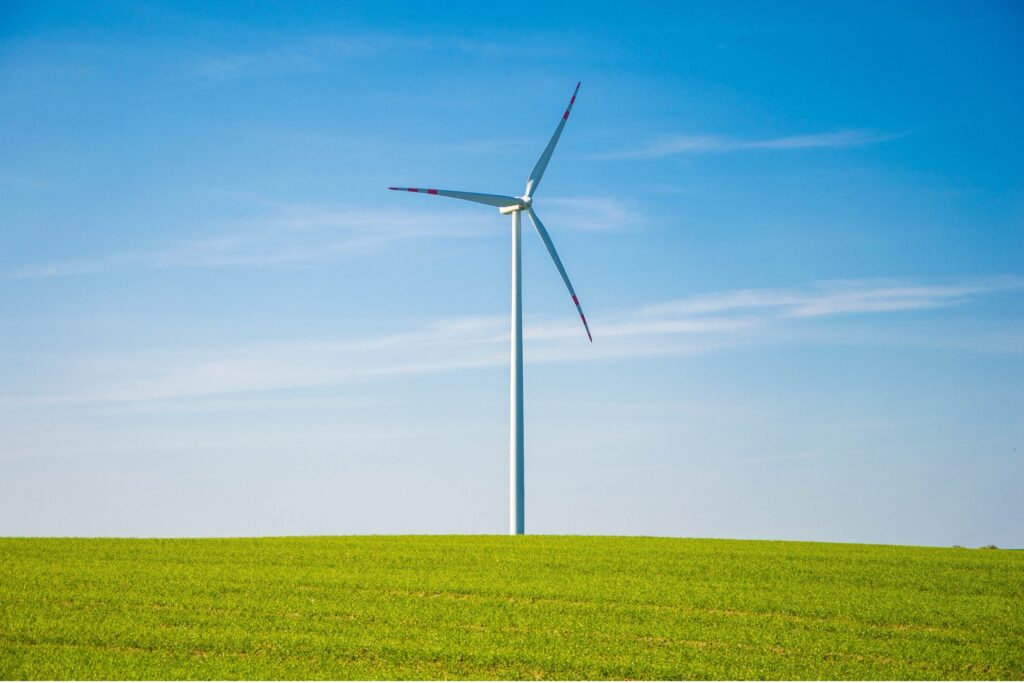
Most of electricity generated in the world comes from burning coal or natural gas, with low emission nuclear, hydro, wind and solar making up the rest of the generation. (I’m writing this from Québec, where electricity comes mostly from hydro.) In other words, for most people in the world, using a kilowatt-hour of electricity is a bit like burning a bit of coal or natural gas. But that’s quickly changing: in 2025, the International Energy Agency shows that the share of low-emission sources is approaching fossil fuels in global electricity generation.
Using coal or natural gas to generate electricity means that producing each unit of electric energy (kilowatt-hours or kWh) costs money: one needs to buy the fossil stuff. In contrast, a common characteristic of low-emission electricity is that the marginal cost of generation and delivery is practically zero.
- Solar, wind, and run-of-river hydro may produce electricity as long as the sun, the wind or the flow of water is available. Turning off generation doesn’t reduce societal costs.
- For reservoir hydro (“big hydro”), it is a bit more complicated. There’s no out-of-pocket cost to open the valves and to generate more, and no savings to turn off. However, generating depletes the upstream reservoir, so there may be an opportunity cost if this energy could be sold at different times for a higher price. However, these hydro plants must maintain minimum flow and managing multiple plants along a river system requires trade-offs, so they aren’t fully dispatchable. Still, marginal operating costs are zero.
- Nuclear plants are often designed to run at a constant power, with ramp-up and ramp-down measured in days. Some newer nuclear plants can better vary their generation, but the only additional cost is that of uranium, which is very small. Reducing output of a nuclear generator doesn’t really reduce costs.
- Finally, the costs of the transmission and distribution grid are also fixed in the short run.
Thus, in a system powered by non-emitting sources, costs are constant in the short run, at least up to its generation or transmission capacity. When demand gets near the system capacity, marginal costs suddenly increase. To balance supply and demand in a constrained system, the system operator will take costly measures such as:
- Dispatching fossil generators, such as natural gas plants, which are costly to run.
- Dispatching grid batteries, buying this power at some previously agreed to rates.
- Importing additional electricity from other jurisdictions.
- Dispatching controllable loads, like HVAC and water heaters for residential customers.
- Paying large industrial customers, like aluminum smelters, to reduce their load.
So, in the end, an additional kilowatt-hour is either “free” (does not add to system costs) or very expensive (near system capacity).
This all-or-nothing characteristic raises a few issues for tariff and market design.
Regulated tariffs are designed to recover the system costs, which only increase during critical peaks, typically a few hours per year. Critical Peak Pricing and Critical Peak Rebate tariffs may therefore be a better signal to customers than fixed Time-Of-Use (TOU) tariffs applied 365 days a year. (Both critical peak and TOU tariffs may be used at the same time, especially when large non-dispatchable generators are present on the system, like in Ontario.) On the other hand, Real Time Pricing tariffs, which vary constantly with market costs, could mean that customers face extremely high prices during peaks, and this can lead to unfairness.
In many jurisdictions, electricity is bought and sold in an open energy market (measured in kWh, a unit of energy) between producers and retailers. The marginal cost of fossil generators set the closing energy market price, with non-emitting producers bidding at zero, knowing that any closing amount above zero is better than nothing. What happens when a system has only (or mostly) non-emitting sources? The closing price remains at zero most of the time. Oops, that’s not good for business. In those cases, a capacity market (measured in kW, a unit of power) may be formed. In a capacity market, producers are paid for the potential capacity they can provide during peaks whether or not their assets are called upon. Hence, more jurisdictions will rely on capacity markets in a low-emission future. Another approach is to get away with markets entirely and go with power purchase agreement between a purchasing agency (or utility) and power producers. Other, mixed approaches may also be found around the world. We are still learning how to best design electricity markets with a non-emitting grid, and this topic is ongoing.
In the end, expect to pay differently for electricity and producers will be compensated differently. At times, electricity prices will be less, but sometime higher, than they are now. This economic transformation is similar in some ways to what happened in telecommunications. Thirty years ago, we paid for each long-distance calls and cell calls, at rates measured in dollars per minute in the case of international calls. Nowadays, we pay a flat fee for the huge bandwidth or large data blocks, and we don’t think twice before doing a FaceTime videoconference with loved ones overseas. Do we pay less for telecom? Well, not really overall, and it’s a lot more complicated, but we get much more out of our money. The same thing will happen with electricity.