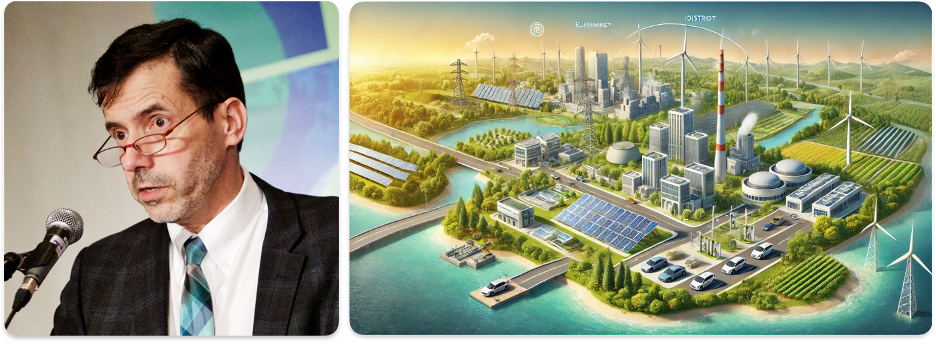
Résumé
Planification de la gestion intégrée des ressources énergétiques (PGIRE) : L’avenir de la transition énergétique du Québec
Le Québec est à un tournant décisif de son évolution énergétique. L’électrification s’accélère et nous devons moderniser notre réseau, intégrer les énergies renouvelables et assurer la sécurité énergétique. Notre Planification intégrée des systèmes énergétiques (PISE) propose une feuille de route pour optimiser les ressources, équilibrer l’offre et la demande et bâtir un avenir durable. Construisons ensemble un système énergétique résilient !
(English Version : https://www.linkedin.com/pulse/integrated-energy-system-planning-iesp-framework-qu%25C3%25A9becs-marcoux-wc95e/)
#TransitionÉnergétique #Durabilité
Introduction : le Québec à la croisée des chemins de l’électrification
Alors que le virage mondial vers la décarbonisation s’accélère, le Québec se trouve à un moment charnière. La province fait face à plusieurs défis, notamment la nécessité de moderniser les infrastructures du réseau, d’améliorer la fiabilité, d’intégrer des sources d’énergie renouvelable croissantes et de répondre à une demande accrue d’électricité dans des secteurs comme les transports et l’industrie.
Les opportunités sont tout aussi nombreuses. En tant que territoire le plus électrifié en Amérique du Nord, le Québec dispose d’une base solide grâce à ses vastes ressources hydroélectriques et à sa grande industrie de fabrication d’équipements électriques. Cette expertise positionne la province comme un leader dans les exportations d’énergie propre. Cependant, les interconnexions limitées avec les états et provinces voisins constituent un obstacle majeur, limitant la capacité du Québec à optimiser le commerce énergétique.
Réaliser une économie durable et électrifiée nécessite une approche de planification robuste et flexible qui aligne l’offre d’énergie, les infrastructures et les besoins émergents. Conscient de ces dynamiques, le Ministère de l’Économie, de l’Innovation et de l’Énergie du Québec (MEIE) du Québec a entrepris l’élaboration d’un plan de gestion intégrée des ressources énergétiques (PGIRE). Ce plan intégré vise à relever ces défis et à saisir ces opportunités, tout en mobilisant un large éventail de parties prenantes pour en assurer le succès. L’acronyme PGIRE sera utilisé dans ce document, même si l’expression planification intégrée des systèmes énergétiques (en anglais: Integrated Energy System Planning, IESP) est plus souvent utilisée dans l’industrie.
Ce document présente ma perspective personnelle sur les PGIRE en général dans le but de contribuer aux discussions en cours et de fournir des perspectives sur les meilleures pratiques et stratégies pour son développement et sa mise en œuvre efficace. Je suis un expert indépendant et je ne suis pas rémunéré par le gouvernement ou Hydro Québec .
Qu’est-ce que la planification intégrée des systèmes énergétiques ?
La planification intégrée des systèmes énergétiques est un cadre stratégique qui coordonne le développement et l’exploitation de systèmes énergétiques interconnectés. En intégrant plusieurs vecteurs énergétiques, tels que l’électricité, le gaz naturel, l’hydrogène et l’énergie thermique dans des secteurs comme les transports, les bâtiments et l’industrie, le PGIRE permet une distribution d’énergie efficace, résiliente et durable. Par exemple, un PGIRE pourrait documenter les scénarios futurs de demande d’électricité dans une ville en pleine croissance, identifier des sources d’énergie renouvelable potentielles comme des parcs éoliens ou solaires pour répondre à cette demande, et planifier la sortie progressive du gaz naturel pour le chauffage, ainsi que les mises à niveau nécessaires des infrastructures de transmission et de distribution. Cela garantit un équilibre entre l’offre et la demande d’énergie, tout en minimisant les risques, les coûts et les impacts environnementaux, et en améliorant la fiabilité et la résilience.
La transition énergétique implique une électrification accrue, reflétant l’importance croissante de l’électricité dans le chauffage, les transports et les processus industriels. Cependant, d’autres sources d’énergie continueront d’être utilisées, telles que le gaz naturel renouvelable et la biomasse pour le chauffage. De plus, pendant les prochaines décennies, les combustibles fossiles continueront à être utilisés, bien que de manière réduite. En intégrant diverses sources d’énergie, technologies et secteurs, le PGIRE garantit que le système énergétique évolue pour répondre aux objectifs sociétaux, économiques et environnementaux.
Le PGIRE diffère de la planification intégrée des ressources (en anglais: Integrated Resource Planning, IRP), souvent utilisée par les services publics électriques pour prévoir et répondre à la demande d’électricité au sein du système électrique. Contrairement aux IRP des compagnies d’électricité, le PGIRE englobe plusieurs services publics et sources d’énergie, met l’accent sur l’efficacité énergétique et souligne une coordination systémique globale. Certains gouvernements, comme la Californie, mènent des « IRP » à l’échelle de l’État qui fonctionnent effectivement comme des PGIRE, ce qui peut prêter à confusion.
Meilleures pratiques dans le PGIRE
La planification intégrée des systèmes énergétiques implique une approche holistique et prospective pour aligner l’offre d’énergie, les infrastructures et les besoins. Pour garantir une mise en œuvre efficace, certaines meilleures pratiques doivent être adoptées :
1. Orientation stratégique
- Mandat clair : Les décideurs définissent une vision stratégique soutenue par des politiques claires, visant des objectifs en matière d’énergie renouvelable, de réduction des émissions, de fiabilité, de résilience, d’accessibilité financière et d’efficacité des coûts.
- Analyse de scénarios : Tester plusieurs scénarios prépare à un large éventail de développements potentiels.
- Adaptabilité : Des mises à jour régulières garantissent que le plan reste pertinent face aux évolutions technologiques, politiques et conjoncturelles.
2. Collaboration inclusive
- Engagement des parties prenantes : Une participation large reflète les priorités sociétales, favorise la confiance et assure la responsabilité.
- Approche interdisciplinaire : Une collaboration intersectorielle évite une planification cloisonnée et favorise des solutions intégrées.
3. Fondations solides
- Décisions basées sur les données : Des modèles de prévision précis garantissent des décisions informées et fiables, particulièrement pour ce qui est des impacts économiques des scénarios.
- Financement et ressources : Des investissements financiers suffisants soutiennent le développement des infrastructures et l’innovation.
- Exécution et supervision : Un suivi continu assure le respect du plan et permet de relever les défis émergents.
En suivant ces pratiques, le PGIRE peut créer un système énergétique durable, résilient et inclusif qui s’adapte aux besoins futurs. Par exemple, le Danemark a réussi à mettre en œuvre des éléments de planification intégrée en combinant l’énergie éolienne avec les systèmes de chauffage urbain, augmentant ainsi l’efficacité énergétique et réduisant les émissions. Alors que le Québec entame son parcours PGIRE, ces principes servent de lignes directrices essentielles pour naviguer dans les complexités de la transition énergétique et assurer un succès à long terme.
Parties prenantes clés dans le PGIRE
Le succès d’un PGIRE repose sur une collaboration efficace entre diverses parties prenantes. Les principaux contributeurs incluent les gouvernements et organismes de réglementation, les services publics, les opérateurs de réseau, les fabricants d’équipements, les leaders de l’innovation, les communautés locales et les groupes de défense. Cet effort collectif garantit que l’expertise issue de divers domaines façonne un système énergétique résilient et inclusif.
1. Gouvernements et organismes de réglementation :
- Les ministères provinciaux, comme le MEIE au Québec, supervisent et régulent le processus de planification.
- Les organismes de réglementation énergétique, tels que la Régie de l’énergie, assurent la conformité et la responsabilité.
2. Services publics et opérateurs de réseau :
- Les services publics, comme Hydro-Québec et Énergir, gèrent la production, la transmission et la distribution d’énergie.
- L’opérateur de systèmes indépendants (Independent System Operators, ISO), lorsqu’il existe, coordonnent la faisabilité technique et la gestion du réseau. Note: il n’y a pas d’opérateur indépendant au Québec.
3. Contributeurs à la connaissance et à l’innovation :
- Les institutions académiques et de recherche offrent une expertise, des analyses de données et des solutions innovantes. Le centre de recherche d’Hydro-Québec (IREQ) pourrait contribuer, surtout s’il s’intègre mieux au tissu industriel du Québec.
- Les experts du secteur privé, comme les cabinets d’ingénierie et de conseil, apportent des connaissances sur les énergies renouvelables, le stockage et les réglementations.
- Les fabricants d’équipements assurent la conception et l’optimisation des composants nécessaires au fonctionnement des systèmes énergétiques.
4. Parties prenantes locales et communautaires :
- Les gouvernements locaux et municipalités adressent les besoins énergétiques spécifiques des villes.
- Les communautés autochtones défendent leurs priorités, leurs droits et leurs préoccupations.
- Les groupes communautaires et le grand public assurent l’adhésion et reflètent les valeurs sociétales.
- Les organisations non gouvernementales (ONG), y compris les associations industrielles et commerciales, enrichissent le processus par des perspectives variées, défendant souvent la durabilité, l’équité et des solutions innovantes qui peuvent aider à combler les fossés entre les communautés, les gouvernements et les industries.
Responsabilité pour le développement et le suivi du PGIRE
Le développement et la supervision d’un plan de gestion intégrée des ressources énergétiques doivent être confiés à une entité indépendante dotée d’une expertise technique, d’impartialité et de solides capacités d’engagement des parties prenantes.
Au Québec, ce rôle a été assumé par le MEIE. Généralement, les ministères gouvernementaux sont bien placés pour diriger les initiatives de PGIRE grâce à leur indépendance, leur représentation étendue des parties prenantes et leur autorité pour répondre aux besoins énergétiques à grande échelle. Cependant, ils nécessitent souvent un soutien technique et opérationnel de la part de cabinets d’ingénierie ou de conseil stratégique.
D’autres entités potentielles pour superviser le PGIRE incluent :
- Organismes de réglementation énergétique : Ces organismes garantissent la conformité et la responsabilité grâce à une supervision indépendante. Toutefois, leur accent sur la régulation peut limiter leur capacité à diriger une planification stratégique.
- Opérateurs de systèmes indépendants (Independent System Operators, ISO) ou organisations régionales de transmission (Regional Transmission Organizations, RTO) : Ces organisations possèdent une expertise technique et opérationnelle solide, mais leur champ d’action se limite souvent aux réseaux électriques, excluant d’autres sources d’énergie.
- Services publics : Les services publics peuvent assumer la responsabilité de la planification à condition de couvrir un large éventail de domaines énergétiques. Au Québec, cependant, aucun service public unique ne domine à la fois les marchés de l’électricité et du gaz naturel, rendant essentiel une collaboration ou un soutien de la part d’entités gouvernementales. Assurer l’indépendance des services publics reste également un défi, particulièrement pour ceux détenus par des investisseurs.
- Consortiums collaboratifs : Les partenariats incluant des gouvernements, des services publics, des institutions académiques et des experts du secteur privé peuvent équilibrer expertise et leadership, mais nécessitent une gouvernance claire pour maintenir le focus et l’autorité.
Résultats attendus et calendrier
Le principal résultat d’un PGIRE est une feuille de route complète pour le système énergétique. Cette feuille de route doit :
- Tenir compte de diverses sources d’énergie : intégrer l’électricité, le gaz naturel renouvelable, l’hydrogène, la biomasse et les combustibles fossiles (en transition) tout en définissant des stratégies pour intégrer les nouvelles technologies énergétiques et éliminer progressivement les sources non durables.
- Répondre aux besoins des communautés : aborder les besoins et priorités énergétiques uniques des différentes communautés, notamment en matière d’accessibilité financière, d’accessibilité physique et de considérations culturelles, particulièrement pour les communautés autochtones et les régions mal desservies.
- Établir une vision à long terme : fournir des prévisions de la demande, des plans de ressources et des stratégies d’investissement alignées sur les objectifs climatiques, tels que la réduction des émissions de gaz à effet de serre et l’amélioration de l’efficacité énergétique.
- Proposer des étapes détaillées de mise en œuvre : offrir des recommandations politiques, des stratégies de mise à niveau des infrastructures, des stratégies de financement et des étapes précises avec des échéanciers pour garantir une exécution fluide.
Cette approche garantit un système énergétique équilibré, inclusif et prêt pour l’avenir. Le processus de PGIRE s’étend généralement sur 18 à 36 mois, selon l’ampleur et la complexité du projet. Les feuilles de route des systèmes énergétiques sont périodiquement révisées pour rester pertinentes et s’adapter aux conditions changeantes. Les mises à jour intègrent les nouvelles technologies, les évolutions du marché, les changements de politiques et les défis imprévus. Les domaines en évolution rapide, tels que l’intégration des énergies renouvelables ou la résilience du réseau, peuvent nécessiter un suivi annuel. Des rapports transparents renforcent la confiance du public et la responsabilité.
Portée géographique
Le plan de gestion intégrée des ressources énergétiques peut être mis en œuvre à différents niveaux géographiques, chacun offrant des avantages et des défis uniques :
PGIRE au niveau de la ville
- Intérêt : adapté pour répondre aux demandes locales en énergie et aux défis urbains, tels que l’électrification des transports ou le chauffage urbain.
- Avantages : solutions personnalisées avec une forte implication communautaire et une gouvernance simplifiée.
- Défis : impact limité sur les systèmes régionaux plus larges.
- Exemple : La Renewable City Strategy (RCS) de Vancouver vise à transitionner la ville vers 100 % d’énergie renouvelable d’ici 2050 dans tous les secteurs, y compris les bâtiments, les transports et les systèmes énergétiques. Voir https://vancouver.ca/files/cov/renewable-city-strategy-booklet-2015.pdf.
PGIRE au niveau régional, provincial ou étatique
- Intérêt : équilibre les ressources énergétiques entre les zones urbaines et rurales, soutenant à la fois les besoins industriels et communautaires.
- Avantages : partage élargi des ressources et renforcement de la résilience grâce à une collaboration régionale.
- Défis : nécessite une coordination entre les municipalités et l’alignement de priorités diverses.
- Exemple 1 : Le plan Integrated Resource Plan (IRP) de la Californie harmonise l’énergie renouvelable et l’intégration urbaine-rurale. Il est mis à jour tous les deux ans pour tenir compte des nouvelles exigences politiques, des objectifs d’intégration des énergies renouvelables et des défis de fiabilité du réseau. Voir https://avaenergy.org/integrated-resource-plan/.
- Exemple 2 : Le plan énergétique à long terme (LTEP) de l’Ontario, révisé en 2013 et 2017, est remplacé par un nouveau plan intégré des ressources énergétiques (IERP) mis à jour tous les cinq ans. Voir https://www.jdsupra.com/legalnews/ontario-charts-its-energy-planning-7798359/. En outre, l’opérateur indépendant du système d’électricité (IESO) publie chaque année une perspective (https://www.ieso.ca/en/Sector-Participants/Planning-and-Forecasting/Annual-Planning-Outlook).
PGIRE au niveau national
- Intérêt : supervise les transitions énergétiques à grande échelle, alignant les politiques nationales sur les objectifs climatiques et la sécurité énergétique.
- Avantages : assure la cohérence des politiques et tire parti des économies d’échelle.Défis : peut négliger les spécificités régionales et les besoins communautaires particuliers.
- Exemple 1 : La stratégie énergétique du Danemark intègre l’énergie éolienne et le chauffage urbain à l’échelle nationale. Cette feuille de route a été révisée plusieurs fois depuis ses débuts dans les années 1970. Voir https://www.irena.org/-/media/Files/IRENA/Agency/Publication/2013/GWEC/GWEC_Denmark.pdf.
- Exemple 2 : Le programme Clean Power 2030 (CP2030) de la Grande-Bretagne vise à garantir que la puisse répondre à ses besoins énergétiques principalement grâce à des sources renouvelables d’ici 2030. Cela inclut l’expansion massive des capacités d’énergie éolienne en mer, solaire, et du stockage par batteries, ainsi que l’extension de la durée de vie des centrales nucléaires existantes. Le programme prévoit des investissements annuels estimés à 40 milliards de livres sterling, ainsi que la construction de 1?000 km de lignes électriques et de 4?500 km de câbles sous-marins. Notons que la production éolienne en Grande-Bretagne se fait principalement au Nord, tandis que la demande d’énergie est plus élevée au Sud, une situation similaire à celle du Québec. Voir https://www.neso.energy/publications/clean-power-2030.
PGIRE au niveau continental ou multinational
- Intérêt : facilite le commerce énergétique transnational, le partage des ressources et le développement des infrastructures.
- Avantages : soutient les projets à grande échelle et la stabilité énergétique régionale.
- Défis : implique une gouvernance complexe et un alignement des politiques transfrontalières.
- Exemple : Le plan de développement du réseau sur dix ans (TYNDP) de l’UE favorise le partage des énergies renouvelables et les réseaux interconnectés. Ce PGIRE transnational est mis à jour tous les deux ans pour refléter les progrès technologiques, les changements dans la demande énergétique et les développements géopolitiques. Voir https://tyndp.entsoe.eu/news/176-pan-european-electricity-transmission-projects-and-33-storage-projects-will-be-assessed-in-tyndp-2024 et https://www.entsog.eu/tyndp.
L’importance de l’exécution
Le succès d’un PGIRE repose largement sur son exécution. Un plan méticuleusement conçu ne peut aboutir sans un chemin clair vers sa mise en œuvre, une supervision robuste et une capacité d’adaptation continue. Les éléments clés d’une exécution réussie incluent :
- Alignement avec les priorités : Les organismes de réglementation des services publics et les autres parties prenantes doivent s’assurer que les investissements sont alignés sur les priorités définies dans la feuille de route du PGIRE. Les actifs non rentabilisés et les projets mal alignés peuvent engendrer de l’opposition, gaspiller des ressources et retarder les objectifs.
- Gestion flexible et adaptative : À mesure que les technologies, les marchés et les politiques évoluent, le système énergétique doit rester flexible. Des mises à jour régulières du PGIRE et l’intégration continue de nouvelles données permettront aux parties prenantes de répondre efficacement aux défis et opportunités émergents.
- Intégration technologique : Exploiter les technologies émergentes qui favorisent la transition énergétique, telles que le stockage d’énergie, les véhicules électriques, l’éolien et le solaire, l’IA, les analyses avancées, la réponse à la demande et les systèmes de surveillance en temps réel, est essentiel. Pour les services publics et les producteurs d’énergie indépendants, ces outils permettent une prévision plus précise de la demande et de la production d’énergie renouvelable, optimisent les systèmes de production (comme l’hydroélectricité) et améliorent l’équilibrage des réseaux. Du point de vue des consommateurs, l’IA peut révolutionner la gestion de l’énergie en optimisant en temps réel le chauffage, la climatisation, la recharge des véhicules électriques, le stockage local et l’éclairage, en fonction des schémas d’occupation et d’utilisation. Ces innovations permettent une collaboration renforcée entre les services publics et les utilisateurs pour atteindre une durabilité accrue, une meilleure efficacité des coûts et une résilience énergétique.
- Coordination des parties prenantes : Une exécution efficace nécessite une collaboration sans faille entre les gouvernements fédéraux et provinciaux, les municipalités, les services publics, les producteurs d’énergie indépendants, les utilisateurs commerciaux et industriels, la chaîne d’approvisionnement en électricité, les acteurs existants des combustibles fossiles et les groupes communautaires. Des canaux de communication clairs et des rôles bien définis sont essentiels pour assurer l’alignement et éviter les erreurs. Cette approche inclusive garantit que toutes les parties prenantes contribuent à une transition énergétique résiliente et efficace.
- Confiance publique et transparence : Des rapports transparents sur les progrès renforcent la confiance du public et assurent un soutien à long terme. Par exemple, la communication transparente du Danemark sur ses projets éoliens a considérablement augmenté l’adhésion publique, accélérant l’adoption des énergies renouvelables et l’atteinte d’objectifs climatiques ambitieux. Les parties prenantes doivent s’engager activement auprès des communautés pour maintenir la responsabilité et garantir l’inclusivité.
- Investissements dans les infrastructures : Des investissements adéquats et opportuns dans les infrastructures critiques, comme les mises à niveau des réseaux, l’intégration des énergies renouvelables et les systèmes de stockage d’énergie, sont essentiels. Ces investissements doivent être planifiés pour gérer la croissance future et les événements météorologiques extrêmes.
Risques d’échec
Les risques qui peuvent mener à une exécution inefficace du PGIRE incluent :
- Défis de croissance : Les services publics nord-américains, qui ont connu une croissance limitée depuis 2000, doivent adapter et étendre leurs opérations par un facteur de 3 ou 4 d’ici 2050 (bien que ce besoin soit moins prononcé au Québec, étant donné son haut niveau d’électrification). Cette transformation exige que la chaîne d’approvisionnement électrique — englobant les fabricants, les prestataires de services professionnels et les développeurs d’infrastructures — se développe également. Cela nécessitera des stratégies innovantes, des investissements substantiels et une coordination complète des parties prenantes.
- Fiabilité et résilience : À mesure que les services publics deviennent le principal système de fourniture d’énergie, ils doivent améliorer la fiabilité (minimiser les pannes). Ils doivent également renforcer la résilience (assurer une récupération rapide et une adaptabilité, particulièrement lors d’événements climatiques extrêmes).
- Rigidité : Une adhésion rigide à des plans dépassés peut conduire à des inefficacités et à des opportunités manquées.
- Sous-investissement : Un financement insuffisant des infrastructures risque de provoquer des pannes, des goulots d’étranglement et des réactions négatives du public.
- Fragmentation des parties prenantes : Un manque de coordination entre les acteurs clés compromet les progrès et gaspille les ressources.
- Inaction retardée : L’hésitation à s’adapter aux conditions changeantes exacerbe les défis existants, retardant les échéanciers pour atteindre les objectifs.
En abordant ces risques de manière proactive et en priorisant l’excellence dans l’exécution, le Québec peut s’assurer que son PGIRE tient sa promesse d’un avenir énergétique résilient et durable.
Recommandations pour le Québec
- Établir un organisme de planification indépendant : Envisager une agence de planification énergétique indépendante pour le Québec, inspirée par des entités comme l’Independent Electricity System Operator (IESO) de l’Ontario.
- Favoriser l’engagement des parties prenantes : Promouvoir l’implication des municipalités, des communautés autochtones et des acteurs du secteur privé peut enrichir le processus de planification en intégrant des perspectives et des expertises diverses.
- Exploiter les forces existantes : S’appuyer sur l’expertise d’Hydro-Québec dans l’hydroélectricité et la gestion des réseaux de transmission ainsi que sur la grande chaîne d’approvisionnement électrique du Québec.
- Se concentrer sur la résilience : Prioriser les mises à niveau des infrastructures pour s’adapter à l’électrification et aux événements météorologiques extrêmes.
- Définir des indicateurs clairs : Établir des indicateurs de performance pour suivre les progrès et adapter les plans si nécessaire.
Conclusion
Le plan de gestion intégrée des ressources énergétiques offre au Québec une feuille de route vers un avenir durable et électrifié, favorisant la résilience énergétique, la croissance économique et la protection de l’environnement. En adoptant les meilleures pratiques, en relevant les défis d’exécution et en alignant les efforts sur les forces provinciales, le Québec peut se positionner comme un leader mondial dans la transition énergétique.